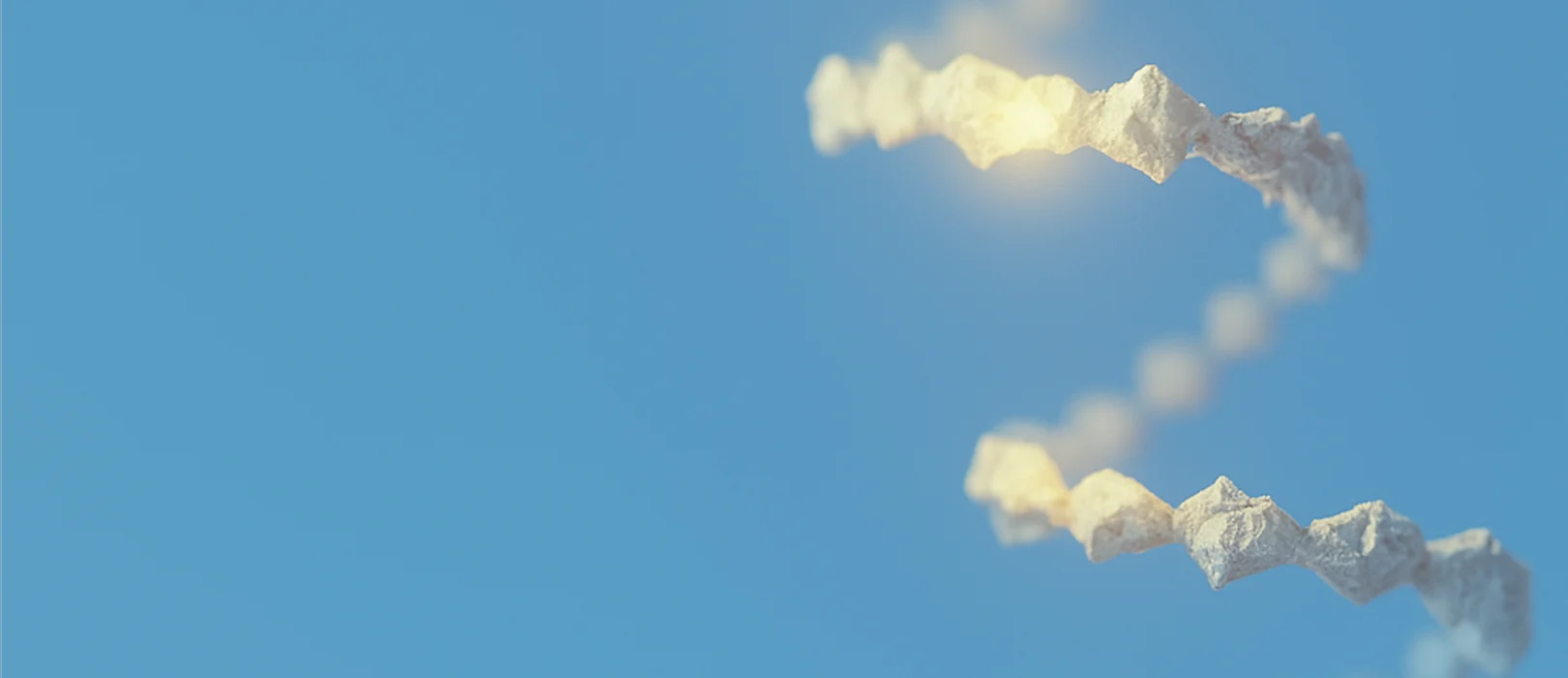
Immunoprecipitation: The Legacy Workhorse of Epitranscriptomics
It is now clear that modifications to both DNA and RNA play a critical role in determining how and when genetic instructions are executed. While DNA modifications have been studied for decades, owing in part to their relatively static and heritable nature, RNA modifications have only more recently become accessible to systematic investigation. This is largely due to the later development of sequencing-based methods capable of detecting RNA modifications that are typically silent with traditional NGS approaches. Unlike DNA modifications, which are relatively stable and passed on through cell divisions, RNA modifications are dynamic and reversible, allowing cells to rapidly adjust gene expression in response to developmental cues or environmental changes.
To date, researchers have identified over 150 distinct RNA modifications across all domains of life, underscoring their evolutionary conservation and regulatory significance. These modifications influence RNA stability, localization, splicing, and translation efficiency1, fundamentally expanding our understanding of RNA beyond its role as a passive messenger to that of a multifaceted regulatory molecule—capable of guiding processes such as catalysis and genome editing. 2 Across all of these contexts—whether structural, catalytic, or regulatory—RNA modifications provide a crucial layer of control, modulating RNA behavior and expanding the functional landscape of the transcriptome. Disruptions in RNA modification pathways have been associated with cancer, neurodegenerative diseases, metabolic disorders, and immune dysfunction.3 If you’re new to this field and want a foundational understanding of how RNA modifications work and why they matter, you might find our beginner’s guide to epitranscriptomics a helpful place to start.
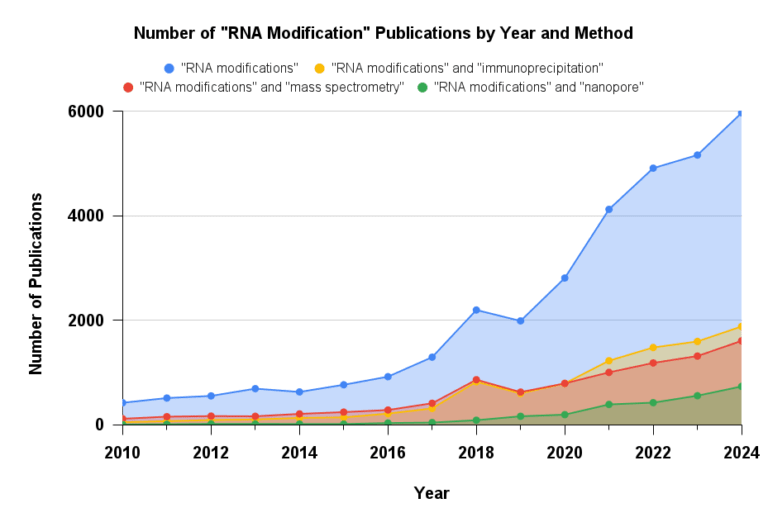
Figure 1: Number of publications that mention “RNA modifications” by year. Research and interest has grown significantly in the last decade. Sourced from Dimensions.ai
This growing interest and significance is obvious when looking at the year-over-year growth in the number of publications that mention “RNA modifications”. In this article, we explore RNA immunoprecipitation (RIP), the most widely used and trusted method for RNA modification detection. We will discuss its role in establishing epitranscriptomics as a field, as well as its limitations as our understanding of the epitranscriptome continues to evolve.
Immunoprecipitation Overview
Figure 2: Typical workflow for RNA immunoprecipitation with antibodies targeting RNA modifications. Antibodies are often immobilized to a surface or solid support before or after incubation with RNA. “Unmodified” RNA fragments are subsequently washed away, while captured fragments are reverse transcribed to generate cDNA libraries for sequencing.
The principle of immunoprecipitation (IP) is straightforward: an antibody raised against a specific RNA modification (such as m6A) is incubated with the target RNA sample, selectively capturing modified fragments. These fragments can then be analyzed using next-generation sequencing (MeRIP-seq), qPCR, or even gel electrophoresis. The simplicity and relatively low cost of IP has made it a widely adopted method and has cemented it as a benchmark for modifications where an effective antibody has been isolated.
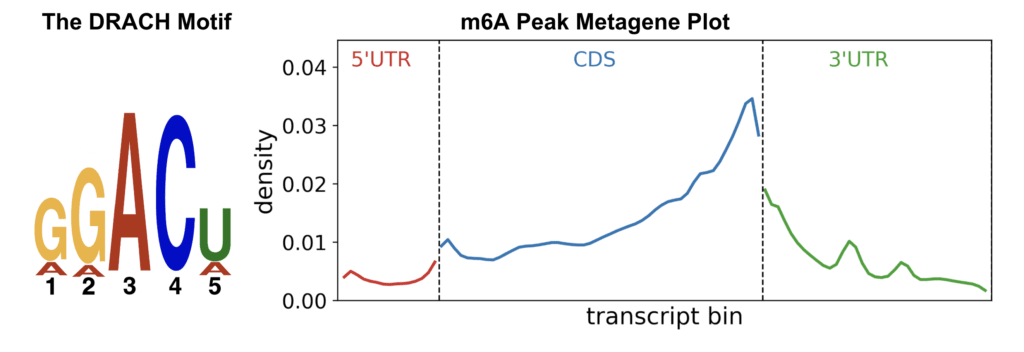
Figure 3: (Top) Representative read pileups enriched using popular anti-m⁶A antibodies. (Bottom) The DRACH sequence motif and peak enrichment at the CDS–3′ UTR junction are hallmark features of m⁶A distribution. Figures are created from publicly available MeRIP data (Zheng et al. 2018)
To this day, immunoprecipitation-based methods remain the most widely used technique in the field, with methylated RNA immunoprecipitation (MeRIP) targeting N6-methyladenosine (m6A) among the earliest and most influential approaches. These initial studies, which focused primarily on m6A due to its relatively high abundance, helped answer several foundational questions. Notably, these seminal papers demonstrated that m6A is enriched within DRACH sequence motifs, particularly near distal exon and 3′ UTR junctions.4,5 In addition, MeRIP was instrumental in identifying and validating the METTL3–METTL14 methyltransferase complex as the core writer of m6A, linking site-specific methylation to a defined enzymatic mechanism.6 Shown above are gene-level examples of RNA fragments containing m6A, enriched using some of the most widely trusted antibodies. These are presented alongside the DRACH consensus motif and a representative metagene plot illustrating the typical distribution of m6A peaks across a hypothetical transcript. These global methylation patterns have since become reference standards, routinely used to validate results in nearly every m6A-related publication where sequence-resolved information is available.
Beyond mapping, MeRIP has also helped illuminate the biological consequences of dynamic methylation. For example, impaired demethylation of m⁶A-modified RNAs has been linked to neurological dysfunction. Deletion of the FTO gene—one of two known m⁶A demethylases—in mice disrupts dopamine receptor regulation, leading to altered neuronal activity and behavioral responses.7 Similarly, dysregulation of ALKBH5, the second m⁶A eraser, has been associated with major depressive disorder.8 Other studies using MeRIP have found dysregulation of m6A in numerous cancers (notably, leukemia), diabetes, obesity, and coronary heart disease.3
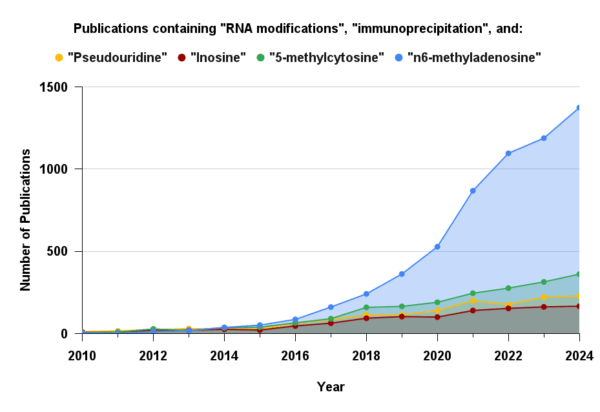
Figure 4: Annual number of publications mentioning both “RNA modifications” and “immunoprecipitation” for the four most stoichiometrically abundant modifications. The widespread adoption of immunoprecipitation for m⁶A is largely driven by the availability of well-validated commercial antibodies. Sourced from Dimensions.ai
A simple literature search using the keywords “RNA modifications” and “immunoprecipitation” highlights the continued prominence of antibody-based enrichment as a core methodology in epitranscriptomics research. For m6A specifically, this reliance is even more pronounced, with nearly half of all m6A publications also mentioning “immunoprecipitation.” However, this reliance is not as strong for other abundant modifications. While multiple antibodies for m6A have been validated and are broadly trusted by the field, the same cannot be said for other modifications, where performance is often inconsistent. Although antibodies for inosine, 5-methylcytosine (m5C), and pseudouridine (Ψ) are commercially available, they are used far less frequently. This discrepancy perhaps reflects a widely acknowledged limitation of the method: the antibody.
The Limitations of Antibody-Based Enrichment
The biggest challenge with RIP is its dependence on antibodies, which are notoriously variable in specificity and sensitivity. While antibodies exhibit higher specificity when binding larger epitopes, such as protein targets, generating antibodies with both high affinity and specificity for small molecules—like a single nucleobase—has long been a challenge.
Shown above are read pileups from a housekeeping transcript comparing antibody-enriched and unenriched control libraries. These examples illustrate off-target binding by two widely used m6A antibodies, which bind highly abundant transcripts regardless of their modification status. Although these antibodies generally exhibit higher affinity for m6A, they still bind unmodified RNA at a significant rate. This non-specific binding effectively siphons off reads from truly modified regions, reducing the overall signal-to-noise ratio. The issue is so widespread that dedicated research groups have developed algorithms specifically to calibrate MeRIP data and distinguish genuine modification signals from background noise.9
RNA’s intrinsic structure presents further challenges for immunoprecipitation-based approaches. Its highly structured and negatively charged nature can limit antibody access, particularly when secondary structure masks modification sites.10 This issue is compounded by the fact that antibodies are typically raised against single nucleobase haptens conjugated to carrier proteins, which do not accurately reflect the structural and electrostatic environment of native RNA.11
The bidentate nature of antibodies also biases fragment enrichment; RNA fragments with dense clusters of the targeted modification tend to be captured more efficiently than those with sparse or isolated sites due to cooperative binding by both arms of the antibody. This enrichment bias contributes to false negatives and can further distort the apparent distribution of modifications across the transcriptome.
Lastly, immunoprecipitation’s accessibility has been a double-edged sword; numerous iterations of MeRIP have been developed, with each lab or group favoring different antibody clones. This variability presents a major challenge for data reproducibility and consistency across the field as antibody clones can vary drastically in specificity and bias. There can be less than 60% overlap in detected modification sites between clones.12,13 These inconsistencies are further complicated by batch effects, polyclonality, variation between antibody lots, and the discontinuation of specific clones used in earlier studies.
Epitranscriptomics Has Evolved. Time to let RIP Rest in Peace?
Although RNA immunoprecipitation has been foundational in shaping the field, its limitations in reproducibility, sensitivity, and specificity are significant, well documented, and should be considered carefully when designing experiments. In most ideal situations, RIP should be paired with proper controls and orthogonal techniques for cross validation which we will discuss further in future blog posts. The need for more sophisticated tools has been recognized by the National Academies with a full length article and call to action. At AlidaBio, we’ve spent the last few years evolving and engineering non-antibody binders that specifically recognize and enrich for RNA modifications with minimal background binding. If this piques your interest, please read our benchmarking study where we directly compare the performance of EpiPlex Binders against the most popular MeRIP antibodies for m6A mapping. As epitranscriptomics advances, so too must the tools we use to study it.
About the Author
Jerome Santos
Jerome Santos holds undergraduate and graduate degrees in Biochemistry, Molecular Biology, and Technology Management from the University of California, Santa Barbara. His academic research centered on the biochemical dissection of intrinsically disordered reflectin proteins, which cephalopods use to construct tunable photonic nanostructures—key to their remarkable ability to dynamically control skin coloration. For nearly a decade, he has been a part of San Diego’s biotech startup community with a focus on bioconjugation, NGS assay development, and molecular barcoding strategies. He currently serves as a Sr. Applications Scientist and Product Manager supporting epitranscriptomics researchers.
References
1. Roundtree, I. A., et al. (2017). Dynamic RNA modifications in gene expression regulation. Cell, 169(7), 1187–1200. https://doi.org/10.1016/j.cell.2017.05.045
2. Cech, T. (2024). The long-overlooked molecule that will define a generation of science. The New York Times. https://www.nytimes.com/2024/05/29/opinion/dna-rna-modern-science.html
3. Jonkhout, N., et al. (2017). The RNA modification landscape in human disease. RNA, 23(12), 1754–1769. https://doi.org/10.1261/rna.063503.117
4. Dominissini, D., et al. (2012). Topology of the human and mouse m6A RNA methylomes revealed by m6A-seq. Nature, 485(7397), 201–206. https://doi.org/10.1038/nature11112
5. Meyer, K. D., et al. (2012). Comprehensive analysis of mRNA methylation reveals enrichment in 3′ UTRs and near stop codons. Cell, 149(7), 1635–1646. https://doi.org/10.1016/j.cell.2012.05.003
6. Liu, J., Yue, Y., Han, D., Wang, X., Fu, Y., Zhang, L., Jia, G., Yu, M., Lu, Z., Deng, X., Dai, Q., Chen, W., & He, C. (2014). A METTL3–METTL14 complex mediates mammalian nuclear RNA N⁶-adenosine methylation. Nature Chemical Biology, 10(2), 93–95. https://doi.org/10.1038/nchembio.1432
7. Hess, M. E., Hess, S., Meyer, K. D., Verhagen, L. A., Koch, L., Brönneke, H. S., Dietrich, M. O., Jordan, S. D., Saletore, Y., Elemento, O., et al. (2013). The fat mass and obesity associated gene (Fto) regulates activity of the dopaminergic midbrain circuitry. Nature Neuroscience, 16(8), 1042–1048. https://doi.org/10.1038/nn.3449
8. Du, T., Rao, S., Wu, L., Ye, N., Liu, Z., Hu, H., Xiu, J., Shen, Y., & Xu, Q. (2015). An association study of the m⁶A genes with major depressive disorder in Chinese Han population. Journal of Affective Disorders, 183, 279–286. https://doi.org/10.1016/j.jad.2015.05.025
9. Li, T., et al. (2024). m6ACali: Machine learning-powered calibration for accurate m6A detection in MeRIP-Seq. Nucleic Acids Research, 52(9), 4830–4842. https://doi.org/10.1093/nar/gkae280
10. Oberdoerffer, S., & Gilbert, W. V. (2025). All the sites we cannot see: Sources and mitigation of false negatives in RNA modification studies. Nat. Rev. Mol. Cell Biol., 26, 237–248. https://doi.org/10.1038/s41580-024-00784-2
11. Feederle, R., & Schepers, A. (2017). Antibodies specific for nucleic acid modifications. RNA Biology, 14(9), 1089–1098. https://doi.org/10.1080/15476286.2017.1295905
12. Zheng, G., et al. (2018). Refined RIP-seq protocol for epitranscriptome analysis with low input materials. PLoS Biol., 16(9), e2006092. https://doi.org/10.1371/journal.pbio.2006092
13. McIntyre, A. B. R., et al. (2020). Limits in the detection of m6A changes using MeRIP/m6A-seq. Sci. Rep., 10(1). https://doi.org/10.1038/s41598-020-63355-3